Post by John Mulrow, Civil Engineering PhD Candidate at University of Illinois at Chicago.
This post describes a chemistry example I use to explain the fundamentals of material and energy flow for sustainability courses at UIC.
A primary task of circular economists is the tracking of material and energy use throughout the production process. One of the simplest ways of doing this is to turn our analysis into a chemical equation. Burning wood to heat the bread oven at Pleasant House is one of the key production processes happening at The Plant. Below I describe how we can represent this process as a chemical equation for conducting a rigorous thought experiment on the possibilities and limitations of circularity.
Like good circular economy accountants we start by making a list of inputs and outputs. In chemistry terms you might say reactants and products. Here is a simple list for the burning of wood:
REACTANTS: wood + oxygen PRODUCTS: energy (heat for baking) + ash + carbon dioxide + water vapor (steam)
Next we need some way of actually accounting for these materials. Chemistry again provides us with the tools. We can represent all of the listed items as a chemical compound so that we can account for the mass of each element involved in the reaction.
Wood, like most fuels (including the food you eat), is basically a pile of interwoven carbon, hydrogen and oxygen atoms. We can use a molecule of glucose (C6H12O6) to represent wood. In actuality wood is mostly cellulose, which are long chains of interlinked glucose molecules. There are many other elements – essential plant nutrients – caught up in the wood. If we add Calcium (Ca) on the reactant side of the equation, representing a key nutritional element, our final wood formula is: C6H12O6 + Ca.
Oxygen, the gas (O2), is our frenemy. It likes to react with or oxidize things, which is how we take its energy into our bodies (via oxidation of hemoglobin in your blood). But it is also constantly reacting with fuels (think forest fires) and tools (think rusty knives and nails). Oxygen is abundant in the atmosphere, so we are constantly benefiting from and fighting against its effects.
Energy, as used in this context, is the release of heat caused by oxidation of the organic compounds in wood. The bonds that hold together the atoms in wood, once broken, release more energy than is needed to recombine them into their product forms. This excess energy is released as heat.
Ash is, nowadays, a very under-appreciated byproduct of wood burning. It is a mixture of all the wood contents not converted to gaseous products during combustion. These are mostly nutritional elements such as potassium, calcium and phosphorus. We will use calcium carbonate (CaCO3), typically the largest component of wood ash, as the representation in our thought experiment.
Carbon dioxide (CO2) and water vapor (H2O) always show up together as products of the combustion of organic fuels. Once the carbons and hydrogens in the fuel are broken up, the energy that held them together is dissipated and they go with the next most stable arrangement, which is to pair up separately with oxygen. Neither Carbon dioxide nor water vapor hold a lot of extractable energy; the reason coal, gas and oil-fueled power plants treat them as non-useful products to be expelled via smokestack. But the fossil fuel economy is no different than the economy of aerobic biology. Humans expel CO2 and water vapor as a byproduct of extracting energy from food!
Here is the final chemical equation, showing that both sides are balanced in quantity of material. The only unbalanced part is the release of energy. Breaking apart the glucose and oxygen molecules releases more energy than is consumed in making ash, carbon dioxide and water, so we only show Energy on the product side of the equation.
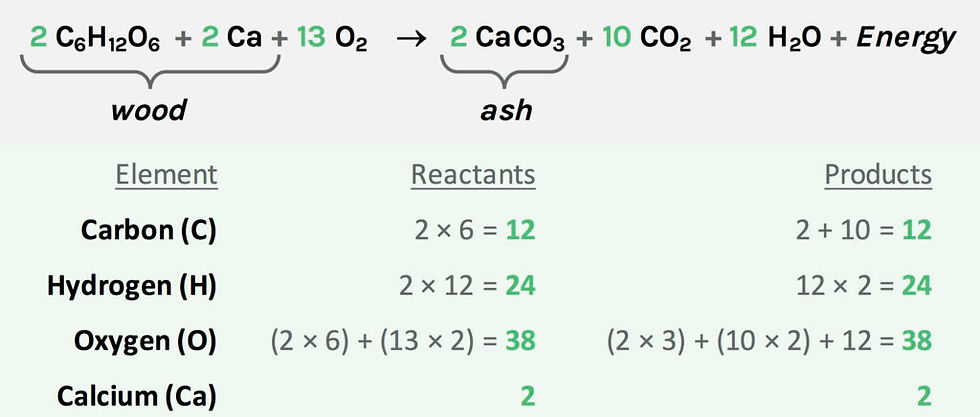
Now we can use this chemical representation to analyze the possibilities and limitations of circularizing the wood burning process.
Possibilities of Circularity
One of the goals of the circular economy is to use the byproducts of one process as inputs in another. Tracking these byproducts at a macro-level was the focus of a material flow analysis conducted in 2016 at The Plant. What are the byproducts in this micro-level case? There is only one product-side item that contributes to baking bread: Energy. Everything else is a byproduct of bread production. There is a chunk of the energy that is technically a byproduct too. This is the energy released by combustion but not imparted to the bread dough. This energy escapes with the gaseous products, heats the bakery air or heats the oven bricks beyond the levels needed for baking bread.
I created this chemical equation example to show how this tool can help in making a full accounting of the inputs and outputs of food production. At Plant Chicago, we have done plenty of brainstorming and experimentation with making the bread-baking process more circular. Here is a quick summary of some of those ideas:
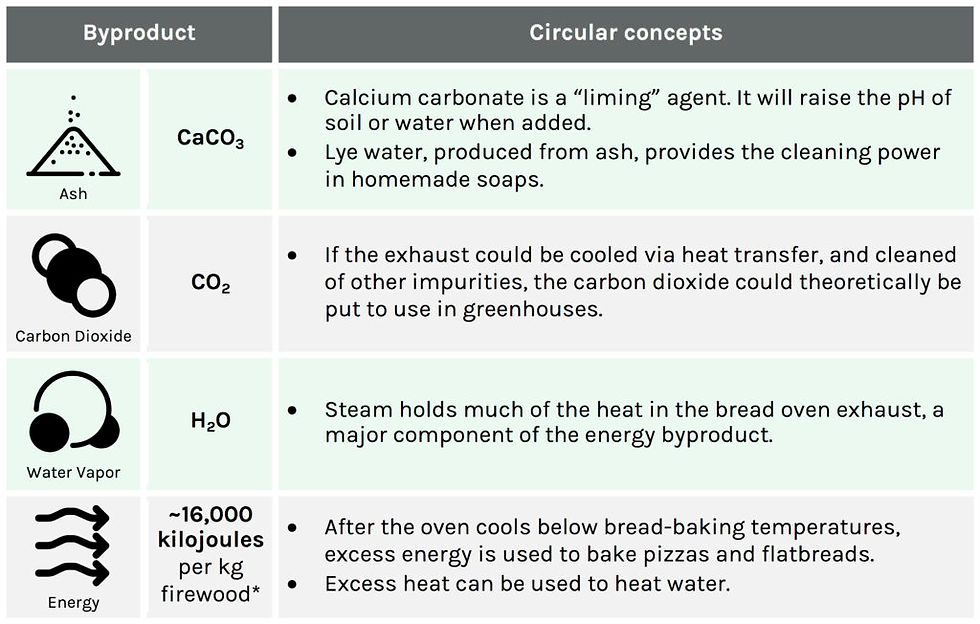
Limitations of Circularity
The next step in making our chemical analysis more rigorous would be to calculate the amount of heat released per mass of wood (glucose) combusted. An estimate is included in the table above, but we don’t really need to go through that process to know that whatever the heat released, we could never capture all of it for doing productive work. Another way to think about this truth is to imagine gathering up all of the carbon, hydrogen and oxygen atoms released from the burnt wood and reassembling the original log so that we might burn it again in an “infinite loop” of wood production and consumption.
Reassembling the molecules is physically possible. There are plenty of labs and companies synthesizing plant-like materials from their organic building blocks, also known as the plastics industry! However, the energy required to reassemble our thought-experiment log is far greater than the energy released from burning it. This is a basic reality of chemistry and physics.
So, while we should certainly strive to get as close to 100% circularity as possible, the Second Law of
Thermodynamics prevents us from ever actually getting there. The Second Law, also known as the Entropy Law, is essential knowledge for circular economists. More on Entropy in future blogs from the Research Steering Committee!
Chemistry and the Circular Economy
The chemical equation approach to material accounting can help in the design of circular solutions for the full array of process inputs and outputs. At the very least, it is a good thought experiment. It can stimulate circular solution brainstorming, reveal new opportunities and expose important thermodynamic limitations.
Comments